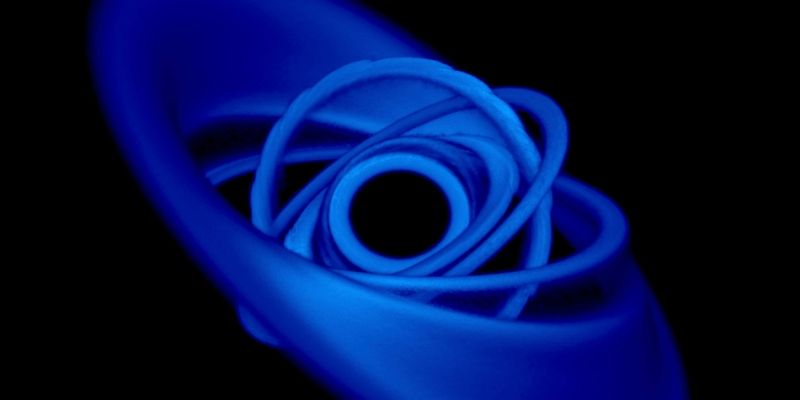
Astronomers are thought to have discovered a new way to measure how fast a black hole spins: by using the wobbly aftermath from its stellar feasting.
The team of international collaborators, including a University of Leeds academic, say their results offer a new way to probe supermassive black holes and their evolution across the universe.
The method takes advantage of a black hole tidal disruption event (TDE), a blazingly bright moment when a black hole exerts tides on a passing star and rips it to shreds.
As the star is disrupted by the black hole’s immense tidal forces, half the star is blown away, while the other half is flung around the black hole, generating an intensely hot accretion disk of rotating stellar material.
Wobble is key
A team of academics and astronomers, led by MIT, has shown that the wobble of the newly-created accretion disk — a flattened, circular or elliptical structure formed when material falls towards a strong gravitational force — is key to working out the central black hole’s inherent spin.
In a study published yesterday (May 22) in the scientific journal Nature, the authors report that they measured the spin of a nearby supermassive black hole by tracking the pattern of X-ray flashes it produced immediately after a TDE. They followed the flashes over several months and determined they were likely a signal of a bright-hot accretion disk that wobbled back and forth as it was pushed and pulled by the black hole’s own spin.
By tracking how the disk’s wobble changed over time, they could work out how much the disk was being affected by the black hole’s spin, and in turn, how fast the black hole itself was spinning. Their analysis showed that it was spinning at less than 25 per cent the speed of light — relatively slow, as black holes go.
They are fascinating objects and the flows of material that we see falling onto them can generate some of the most luminous events in the universe.
Dr Chris Nixon, Associate Professor in Theoretical Astrophysics in Leeds’ School of Physics and Astronomy, co-authored the paper. He said: “These findings are really exciting for future studies into black holes and their evolution.
“The spin of a black hole tells you about its history, and the method we have developed — of measuring its spin speed — will help us to deepen our understanding about how black holes evolve.
“They are fascinating objects and the flows of material that we see falling onto them can generate some of the most luminous events in the universe. While there is a lot we still don’t understand, there are amazing observational facilities that keep surprising us and generating new avenues to explore. This event is one of those surprises.”
Greater understanding
Dr Nixon, whose areas of expertise include accretion disc physics, black holes and TDEs, contributed to the interpretation of the event and the theoretical models to provide an explanation for the behaviour observed.
He added: “These tidal disruption events are great for testing our understanding of how matter flows around black holes.This is an important topic in astrophysics as the energy generated by black holes can have a strong impact on their surroundings.”
The study’s lead author, MIT Research Scientist Dheeraj “DJ” Pasham, said the new method could be used to gauge the spins of hundreds of black holes in coming years. If scientists can survey the spins of many nearby black holes, they can start to understand how the gravitational giants evolved over the history of the universe.
Co-authors include collaborators from institutions including NASA; Masaryk University in the Czech Republic; the University of Syracuse; Tel Aviv University and the Polish Academy of Sciences.
Shredded heat
Every black hole has an inherent spin shaped by its cosmic encounters over time. If a black hole has grown mostly through accretion — brief instances when some material falls onto the disk — this causes it to spin at quite high speeds. In contrast, if it grows mostly by merging with other black holes, each merger could slow things down as one spin meets the other.
As a black hole spins, it drags the surrounding space-time around with it. This drag effect is an example of Lense-Thirring precession, a longstanding theory that describes the ways extremely strong gravitational fields, such as those generated by a black hole, can pull on the surrounding space and time. Normally, this effect would not be obvious around black holes, as they emit no light.
However, in recent years physicists have proposed that in instances such as a TDE, scientists might have a chance to track the light from stellar debris as it is dragged around. Then, they might hope to measure the black hole’s spin.
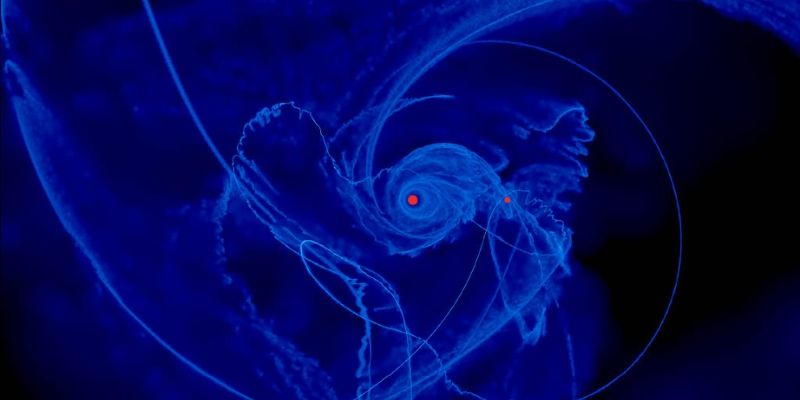
During a TDE, experts predict that a star may fall onto a black hole from any direction, generating a disk of white-hot, shredded material that could be misaligned to the black hole’s spin. The accretion disk can be imagined as a tilted donut spinning around a donut hole that has its own, separate spin. As the disk encounters the black hole’s spin, it wobbles as the black hole pulls it into alignment. Eventually, the wobbling subsides as the disk settles into the black hole’s spin plane. Scientists predicted that a TDE’s wobbling disk should therefore be a measurable signature of the black hole’s spin.
Pasham, a member of MIT’s Kavli Institute for Astrophysics and Space Research, explained that the key to this would be to have the right observations.
“The only way you can do this is, as soon as a tidal disruption event goes off, you need to get a telescope to look at this object continuously, for a very long time, so you can probe all kinds of timescales, from minutes to months.”
Flash of light
For the past five years, Pasham had looked for TDEs bright enough, and near enough, to quickly follow up and track for signs of Lense-Thirring precession. In February 2020, the team got lucky, with the detection of AT2020ocn, a bright flash, emanating from a galaxy about a billion light years away.
It appeared to be the first moments following a TDE. Being both bright and relatively close, Pasham suspected this might be the ideal TDE in which to look for signs of disk wobbling, and possibly measure the spin of the black hole at the host galaxy’s centre.
Pasham said: “The key was to catch this early on because this precession, or wobble, should only be present early on. Any later, and the disk would not wobble anymore.”
The team discovered that NASA’s NICER telescope was able to continuously observe the TDE for months at a time. NICER — an abbreviation for Neutron star Interior Composition ExploreR — is an X-ray telescope on the International Space Station that measures X-ray radiation around black holes and other extreme gravitational objects.
Dr Nixon and his colleagues looked through NICER’s observations of AT2020ocn over 200 days, finding that the TDE emitted X-rays that appeared to peak every 15 days, for several cycles, before eventually petering out. They interpreted the peaks as times when the TDE’s accretion disk wobbled face-on, emitting X-rays directly toward NICER’s telescope, before wobbling away while continuing to emit X-rays - similar to waving a flashlight toward and away from someone every 15 days.
The researchers took this pattern of wobbling and worked it into the original theory for Lense-Thirring precession. Based on estimates of the black hole’s mass, and that of the disrupted star, they came up with an estimate for the black hole’s spin — less than 25 percent the speed of light. It is believed to be the first time scientists have used observations of a wobbling disk following a TDE to estimate the spin of a black hole.
Further Information
The paper, Lense–Thirring precession after a supermassive black hole disrupts a star, was published in Nature on Wednesday May 22.
DOI: https://doi.org/10.1038/s41586-024-07433-w
This research was funded, in part, by NASA and the European Space Agency.
Dr Nixon was part-funded by the Science and Technology Facilities Council (STFC) and the Leverhulme Trust.
Main picture caption: Snapshot of a simulation of a disk that is misaligned to the spin of a black hole. It shows the disk structure which displays rings of gas that are precessing due to the rotation of the black hole (the Lense-Thirring effect). (Nixon et al. 2012: https://ui.adsabs.harvard.edu/abs/2012ApJ...757L..24N/abstract)
Embedded picture caption: Snapshot of a simulation of a tidal disruption event in a supermassive black hole binary system. The streams of stellar debris interact with both black holes (red dots) and wrap around the rotating binary system. Disks are formed around each of the black holes, and these disks transport the debris into the black holes. (Coughlin et al. 2017: https://ui.adsabs.harvard.edu/abs/2017MNRAS.465.3840C/abstract)
For media enquiries, please contact Deb Newman in the University of Leeds press office via d.newman@leeds.co.uk.
This article was adapted from MIT News.