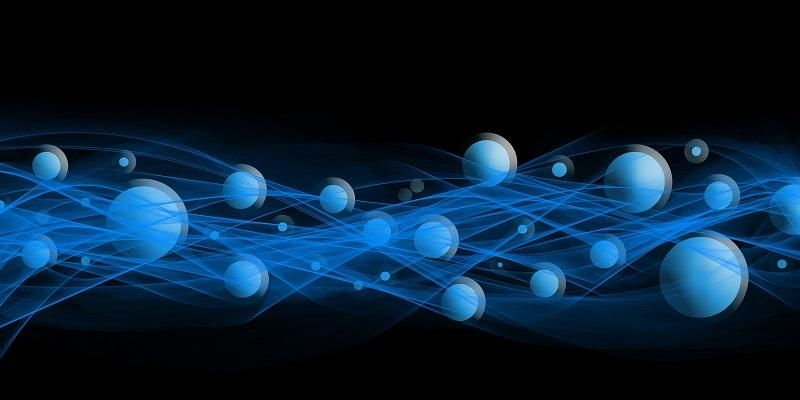
Researchers have come up with a theory to explain one of the mysteries at the heart of quantum physics.
Scientists from the Theoretical Physics Research Group at Leeds have modelled why atoms in a quantum state defy one of the fundamental laws of classical physics - when energy is applied to matter, atoms reorder themselves to find a new equilibrium.
An example of that transformation is seen when a gas is put under pressure and it reduces in volume.
Their hypothesis could also provide a solution to one of the big technical challenges with the development of quantum computers: the need to keep them at very low temperatures.
...it opens up the possibility that people can exploit these quantum properties to store and manipulate data.
Dr Zlatko Papic
The new theory, developed in collaboration with colleagues in the US and Europe, is reported in two separate papers in the journal, Physical Review Letters.
Christopher Turner, a doctoral student in theoretical physics at Leeds and lead author of the first paper, said: “Our research is changing our understanding of quantum systems.
“We have known that individual atoms display an angular momentum which is known as spin. But what we believe is that it can also exist in a system made up of groups of atoms where there is co-ordinated spin between all the atoms.
“An atom seems to be aware of what the atoms around it are doing. A simple analogy would be to think of the atoms taking part in an elaborate dance.”
The theory is built on a belief that each atom is sensitive to the behaviour of atoms around them and they form what is known as a correlated system where the behaviour of the system cannot be understood by examining individual atoms.
The study is building on research from a team at Harvard University and the Massachusetts Institute of Technology that developed a quantum computer from 51 Rydberg atoms, atoms that had been put into a high energy state.
Atoms involved in a 'correlated dance'
When energy was applied to the atoms they oscillated for a considerable period of time before eventually returning to their original stable state. They too did not find a new equilibrium, in contradiction of the view of classical physics.
Kieran Bull, also a doctoral student in theoretical physics at Leeds, was lead author in the second paper in Physical Review Letters.
He said: “We were excited by the elegance of the results described in the first paper. We wanted to understand how a robust memory of a quantum state at high temperatures could be generalised to a wider class of systems.”
The idea of a ‘memory’ in quantum physics describes how the atoms are able to return to their initial state, despite being in a chaotic state.
The researchers found many examples of systems which also participate in a correlated dance, but their dancing pattern is so complex that it cannot be described as a simple spin bouncing between its available states.
Potential practical applications
Dr Zlatko Papic, from the Theoretical Physics Research Group at Leeds, believes there is a practical application of this newly-identified spin process in the development of quantum computing.
Quantum computing harnesses quantum mechanics to bring about a massive increase in processing power.
In traditional computing, information is stored in bits that are coded as zero or one.
In quantum computing, information is coded in qubits and the angle or state of the atomic spin. Because more permutations are possible, the amount of data that can be held and the speed at which it can be manipulated by the system is much larger.
Dr Papic added: “Our research is exciting. It shows there are new quantum effects which allow for protracted oscillations to occur when energy is applied to Rydberg atoms.
“On a practical level, it opens up the possibility that people can exploit these quantum properties to store and manipulate data.
“One of the problems scientists face at the moment is that quantum devices have to be kept very cool because the data they hold is corrupted by high temperatures.
“That is not a problem with this system. It is very robust.”
Further information
For more information, please email David Lewis at d.lewis@leeds.ac.uk